Air-to-Water Heat Pump Retrofit
A mechanical engineer shares lessons learned after replacing his propane boiler with an air-to-water heat pump to heat his home.
By Brian Just
Air-to-water heat pumps generate a lot of buzz, but is anyone installing them? When we purchased our hydronics-based home in 2015, I was intrigued by the prospect of replacing the propane boiler with an air-to-water heat pump (AWHP). But no one was doing it and the equipment options were limited: the Daikin Altherma had been pulled from the market, the Sanden was being tested at a few homes in the Pacific Northwest, and I didn’t know of any other market-ready options.
Four years on, we finally took the plunge and are now two-thirds of the way through our first winter with our AWHP. I’ve learned a lot. Given how few stories are circulating about this technology, I figured I’d share ours. Before I dive in, I’ll note that many feel the industry and contractor base isn’t there yet. So, is it? I now think the answer is “yes” or at least “pretty much.”
Project goals
During our first year in the house, we had a carbon monoxide scare that evacuated us from our home in the middle of the night. It was a leak issue easily fixed by a boiler service, but reinforced our desire to wean the house from propane completely: not only would it improve health and safety, we’d never again have to deal with propane supply contracts, winter fuel deliveries, and volatile unregulated fuel prices (currently, propane costs are low, but hovered in the $4/gallon range in recent years). Given the relatively clean generation portfolio of our electrical utility, transitioning from fossil fuel combustion to electric could also help us be better climate citizens.
We could have just installed minisplits—it certainly would have been easier, as I’ll get to—but we liked our radiant slab and wanted to reuse at least some of our hydronic infrastructure, and we didn’t want minisplits heads and linesets scattered around the interior and exterior of the house.
In sum, we wanted to eliminate fossil fuel combustion in the home, improve health and safety, use existing hydronic infrastructure, be pinned to more stable energy prices, and run our home with lower greenhouse gas impacts. There were other benefits realized along the way, but I’ll get to those later.
Fixing Distribution
Our slab-on-grade home has radiant heating tubing embedded in the slab for the main level. The second-floor zone consisted of baseboard radiators installed in series (a single loop) serving four rooms. To evaluate the system, it was critical to not only figure out the home’s heating design load, but to calculate room-by-room loads. I used REM/Rate to get an estimate of the heating design load, and then an Excel-based UA calculator for a deeper dive and to look at the room loads.
On doing this, I found that the existing system was 30% undersized in one upstairs room—the master bedroom, which was not a surprise given our comfort experience. And that’s with 180°F supply-side water from a boiler. Now, substituting in 120°F supply water—which is a reasonable upper temperature limit for an air-to-water or ground-source heat pump—the existing emitter was about 4x undersized. The other rooms upstairs would be 3x to 4x undersized with the lower temperature supply.
This part is frustrating: If the second floor of my house had been plumbed in parallel home runs (dedicated supply and return from the mechanical room to each upstairs room), upgrading heat emitters could have been relatively straightforward. But in series, it’s was pretty much impossible to get adequate heat to the second, third, and fourth rooms in the loop after starting with 120°F water and dropping degrees every time the water hits another emitter.
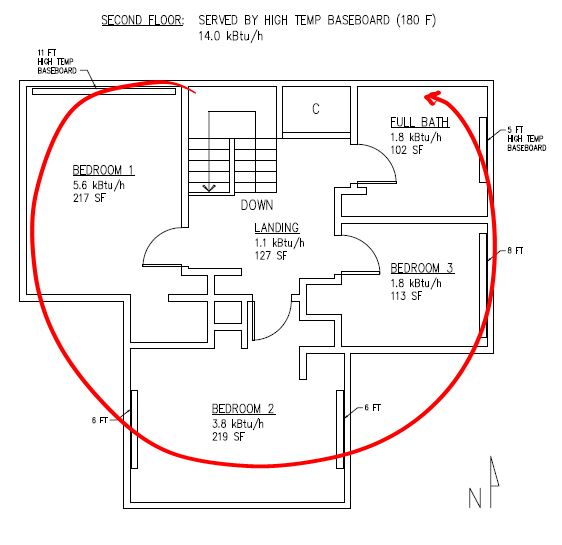
The right way to do this was to get home runs to each room, but that meant ripping open a combination of floors, walls, and ceilings. This was a major barrier that we only justified (eventually, and not without major reservations) after committing to install an ERV and its duct work at the same time as the heating system work. And while we were busy ripping things apart, we decided to gut renovate the only full bathroom in the house. Scope creep? Absolutely. But these other factors were ultimately what helped justify moving forward with the project rather than just installing a gaggle of minisplits, dropping the idea of balanced ventilation, and calling it a day.
Onward. The new distribution system had to be properly designed. In 2016, I took a John Siegenthaler course on AWHPs and got some decent foundational knowledge on designing low water temperature distribution systems. People who work with ground-source heat pumps already know the options: radiant walls/ceilings/floors, high output baseboard, fan coil units, fan-assisted panel radiators, and just plain panel radiators.
I ultimately settled with panel radiators. They’re ubiquitous in other parts of the world, are mechanically very simple, don’t require power, and they’re relatively inexpensive. My room loads for upstairs varied from 1800 btu/h to 5600 btu/h. The final design included two large three-plate panel radiators (for the two larger bedrooms), two smaller two-plate panel radiators (for the bathroom and third bedroom), and a tiny two-plate radiator for a loft space.
It’s worth noting that someone accustomed to boiler installs would consider them grossly oversized. But for 120°F supply, a factor of about 0.28 has to be multiplied by the manufacturer listed output (at 180°F supply)—though they are perfectly capable of putting out useful heat at lower temperatures, most manufacturers don’t publish the output that low and you have to dig into technical literature to find it. Note that the large size of the radiators wasn’t a problem; hundreds of sizes are available, which allowed me to get creative in matching panel radiators to the unique space and window configurations of each room. And interestingly, selecting larger sizes didn’t come at a significant price premium.
Selecting the AWHP
At my home’s outdoor heating design temperature (-6°F) and an indoor temperature of 68°F, REM/Rate gave a design heating load of 31.1 kbtu/h. My own UA spreadsheet yields 32.3 kbtu/h.
Currently, I’m aware of five residential-sized air-to-water products available in the US: Aermec, Arctic, Chiltrix, Nordic, and SpacePak. All use R-410a refrigerant. Sanden makes an air-to-water heat pump with CO2 as its refrigerant, which was very appealing from a global warming impact perspective, but currently only markets a system for supplying domestic hot water (DHW); a modulating space heating version is being tested in the Pacific Northwest but is not available for sale.
I liked certain features of a split system, which narrowed things down to one manufacturer, Nordic. Though not familiar with installs using that equipment, I was comforted that they were a North American manufacturer (New Brunswick, Canada) with a product built around their long-established ground source heat pump line. Advantages of their system vs. a mono-bloc design were that it keeps most sensitive electronics and moving parts indoors, doesn’t require pan heat, crankcase heat, or constant circulation, and avoids the need for glycol, as only refrigerant passes through the building envelope. There are advantages to the mono-bloc design as well, which I’ll cover later.
All of Nordic’s units operate down to -6°F, though at low temperatures (5°F and lower, from my understanding) it will only heat water to 105°F. Right-sizing was important to me and I settled on the ATW-65 model; at 5°F, its capacity matches almost exactly my heating load calculation at that temperature.
Their heat pump is designed to integrate with a EcoUltra buffer tank (50 or 70 gallon options) with an electric coil to provide backup in case the heat pump is out of commission, can’t meet the set point, or the temperature drops below the operation limit. I chose the 70-gallon version and an appropriately sized coil. For what it’s worth, published heat pump performance suffers a lot at 5°F and below; the reality is we’ll mostly burn wood when it’s that cold out.
System design
Early iterations of my design (a couple years ago) were based on a mono-bloc heat pump and circulating 30% glycol through the whole system. As mentioned above, I liked the split system in part for the reason that I could avoid having to think about antifreeze and its maintenance.
Some other decisions we made:
- Cooling: we opted against it; I didn’t want to deal with condensate lines and neither my wife nor I like A/C
- Domestic hot water: after considering various viable ways to integrate it (the Nordic has built-in provision for DHW preheat), I decided to keep DHW independent
- Piping: we decided on 3-pipe design for its direct-to-load pathway with less mixing in the buffer tank
My credo was simplicity, simplicity, simplicity, which also influenced decisions to leave cooling and DHW out of the picture. The result, I think, is mechanically elegant and repeatable. Many thanks to John Siegenthaler, who was a great thought partner and did the system schematic, and Nathan Mascolino of Efficiency Vermont, who is an incredibly knowledgeable advisor. (Disclosure: I work with Nathan at Efficiency Vermont.)
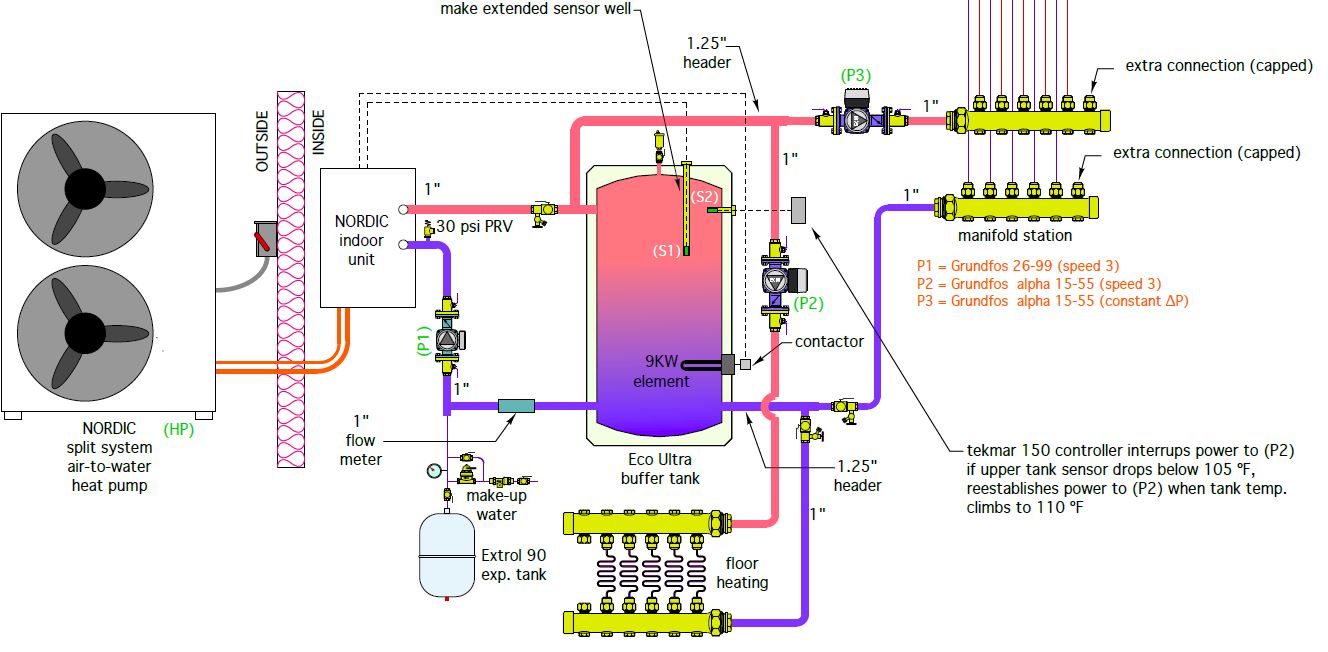
Installation
Phase I of the project occurred in April-June 2018, when I selectively tore up the house interior to put in the new distribution system for the heating and ventilation systems (and redid the master bath). It was painful and took longer than we hoped. Is anyone surprised?
I used PERT (polyethylene raised temperature) tubing rather than PEX (cross-linked polyethylene). This stuff will only ever see temperatures up to 120°F and PERT can handle 180°F, so there was no need for PEX. There are advantages to both materials, but I liked that PERT is more easily recyclable and the cost difference was not significant.
After routing the distribution from the mechanical room and into the office (losing two small storage nooks), the distribution went up a level to serve the master bedroom. These two rooms both had to be padded out 1 1/2 inches to make room for the tubing to avoid a structural beam. A portion of the distribution then went up another level into a loft space and crossed into an unconditioned flat attic (covered with R-30 of air sealed insulation), before dropping down an interior wall and into the floors of three more rooms. This was all very inconvenient, but worked out well in the end and no one who sees the home would ever know.
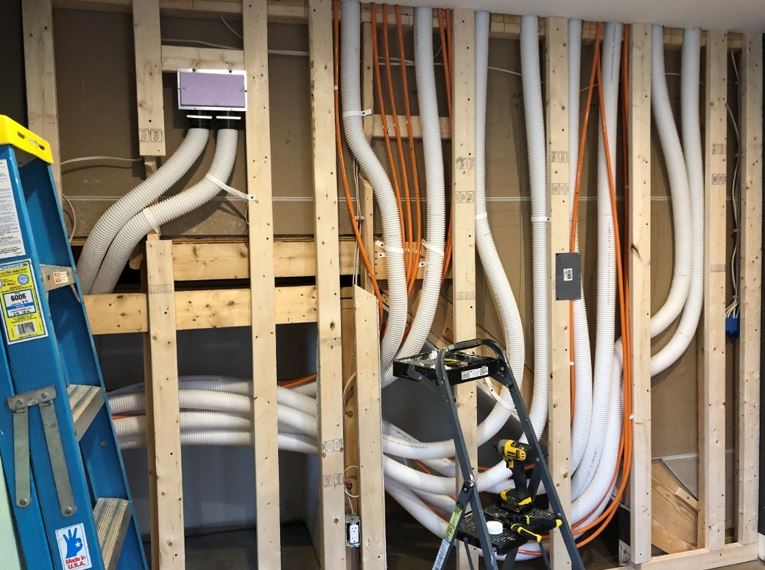
The project paused for nearly a year at this point. We left the boiler in place to serve the downstairs through the 2018-19 winter. We burned wood to keep the upstairs warm enough. We sleep on the cool side, so this was acceptable for one winter (and one winter only).
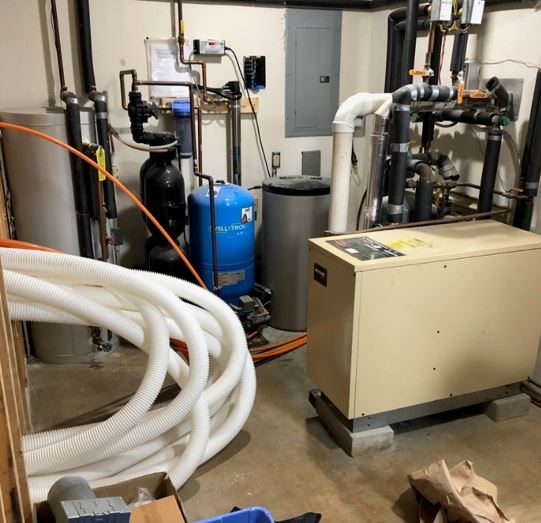
Phase II occurred April-June 2019. The boiler and indirect tank were extracted, our propane tank was disconnected and dug out of the front yard, and we put in a heat pump water heater to have continuity in DWH. The air-to-water heat pump installation then took place.
I was fortunate to work with a quality-focused and conscientious HVAC contractor Bill Chidsey of Solar Harvester throughout. We worked together to put in piers below the frost line for the outdoor unit and prep the indoors. He was diligent with his handling of ACR tubing (cut, ream, min. 15% silver, dry nitrogen purge, vacuum), which is critical in order to avoid refrigerant contamination, minimize future leaks, etc. This is where a mono-bloc system would shine—you avoid refrigerant lines altogether and the necessary precautions including great care taken to minimize risk of flexing and damaging joints in the long term.
Timewise, I was under pressure to get the job done (my wife was pregnant with twins), so it took some active management to coordinate the sequencing of heating, ventilation, and electrical work that had to take place. But it all worked out. I got the Zehnder ventilation system in and commissioned. Bill’s copper work in the mechanical room was impeccable and we put in a new manifold for the ground floor that would allow me to balance the highly variable ground floor slab loop lengths. An identical one was used for upstairs as well; these are compatible with zone valves if needed/wanted in the future, and each has an extra connection—for example, to facilitate installation of a small emitter if it’s determined in the future that the heat pump water heater over-cools the mechanical room.
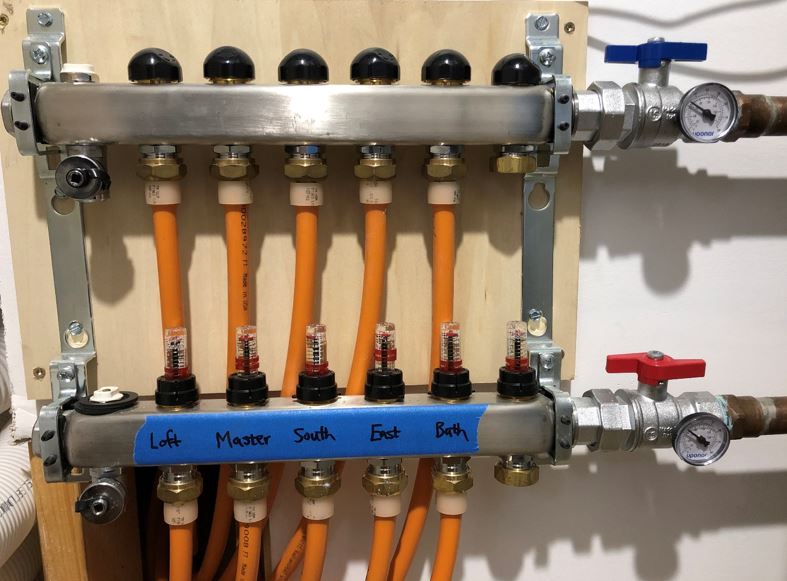
Commissioning went pretty smoothly, though an error in Nordic’s instructions on the buffer tank setup cost a fair bit of time to troubleshoot (hopefully the manual has been updated by now). When we needed to contact their technical support, they were responsive.
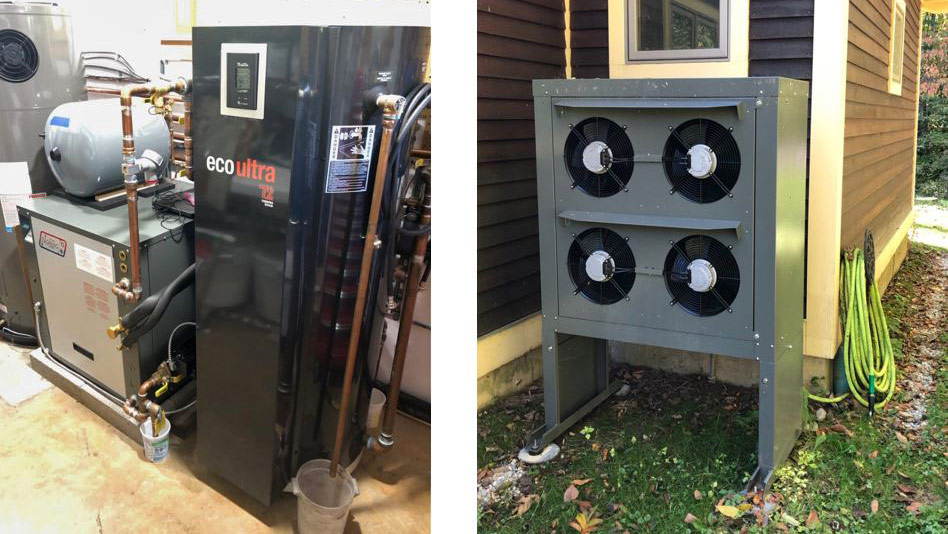
Operation
The system is working well. Operation is straightforward: all the heat pump does is heat water whenever the buffer tank falls below a delta-T on the setpoint. The downstairs zone is controlled by a programmable thermostat—we chose EcoBee largely for its online portal that allows downloading of temperature and relative humidity data. A call for heat activates the single Grundfos smart circulator pump (set to constant flow) for the radiant slab loops.
The upstairs zone is also controlled by a programmable thermostat located in the mechanical room, but I’m not using it in a conventional way. I began the winter with it set to 80°F at night (when we want heat upstairs) and 50°F during the day (when we don’t need it)–basically functioning like an on-off switch on a timer. Each of the five panel radiators has a thermostatic radiator valve (TRV), and we have them dialed in to the temperatures we want: two of them are set to stay in the 50s, two keep rooms at 60°F, and the room where we all sleep is set to 68°F. This will of course change as our family grows and we start using more rooms. The TRVs are pretty slick (for more on how they work, this is a good primer). This zone is served by a single Grundfos smart circulator pump (set to constant delta-P) that adapts flow based on what the panel radiators are calling for. It’s a nice way to minimize both control complexity (i.e. things that can go wrong) and circulator electricity.
The Nordic has integral outdoor reset, which allows you to reduce buffer tank setpoint with warmer outdoor temperatures/lower building load in order to maximize COP.
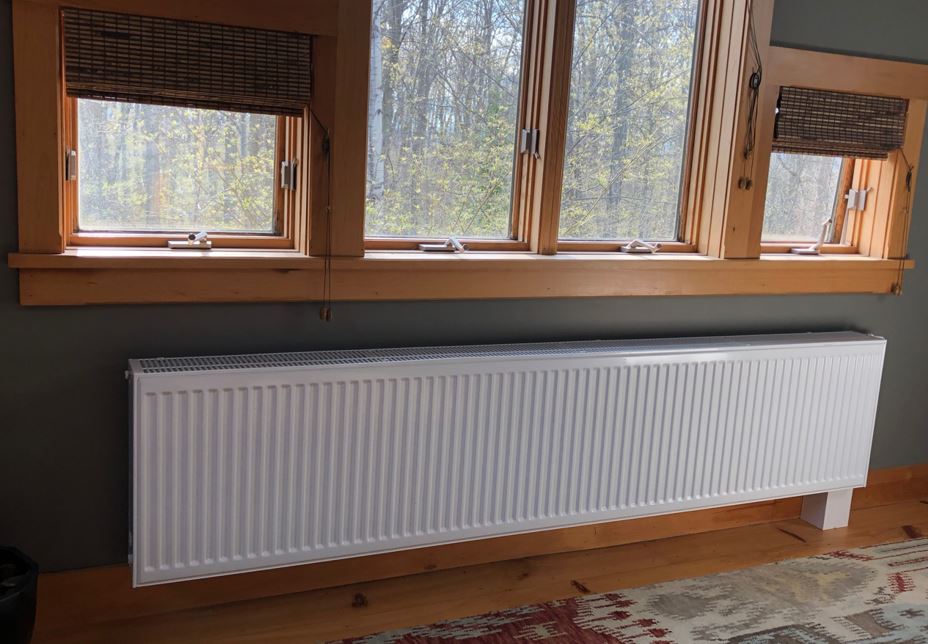
One of the things I like about the design is how it minimizes extra electrical loads. As I mentioned earlier, the design of this heat pump means no constant circulation, no pan heater, no crankcase heater—items that could potentially chew up hundreds of watts 24/7. The circulator pump for the downstairs zone draws about 15W or 40W, depending on which constant speed setting I’m using (still playing with this). The upstairs circulator pump electricity use varies from about 15W-35W.
Performance
The system has a monitoring system installed: current transformers on circuits, a high accuracy turbine flowmeter on the heat pump-buffer tank loop, and some temperature sensors both integral to the heat pump and on the distribution.
The system is operating at a COP of about 3.0 at 30°F. At higher temperatures it approaches 4.0 while it dips below 2.0 when temperatures hit the single digits. My seasonal COP from November to mid-January is 2.49, which includes defrost energy.
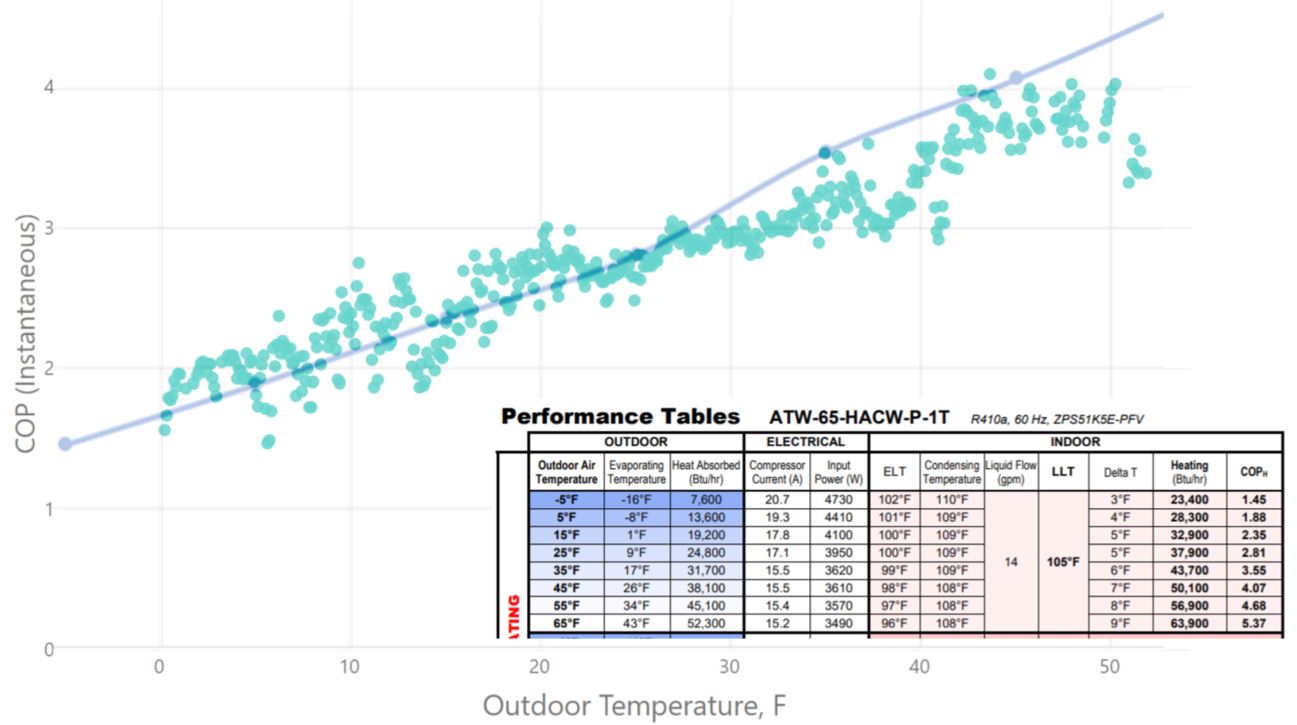
My results closely align with the manufacturer specs and is higher than published data I’ve seen (so far) on other residential air-to-water systems. We’ve had temperatures drop below zero and it’s performing well. In fact, I’ve found that our distribution system works just fine thus far with 105°F supply water, which indicates that my heat load calculations (sized emitters assuming 120°F supply) were likely on the conservative side.
Later, I’ll process data from the entire winter and will look at total cost of this winter’s heat (new system vs. old system at current propane price), greenhouse gas impacts using 2018-19 ISO New England fuel mix data (new system vs. old system), and other items. I presented some of this—along with an overview of the project economics and several dozen photos—at the Better Buildings by Design conference in Burlington, VT in early February 2020 and will be doing a similar presentation at Building Energy Boston in late March.
For those exclusively tuned into payback, note that installing one of these is probably a big leap for an existing home, even with the generous $1000/ton rebate from (and currently still provided by) local efficiency utility, Efficiency Vermont. For new construction, I don’t think the case is hard to make. In most existing buildings, retrofitting the distribution system is a major hurdle; most of my pain (and expense) could have been eliminated had the original contractor put in home runs to the upstairs rooms.
In my opinion, anyone putting in a hydronic distribution system designed for 180°F is doing customers a huge disservice in terms of future retrofit-ability. Even if putting in a fossil fuel system today, consider:
- Install a home run to each emitter (or a supply and return loop with zone valves at the take offs)
- Size the heat emitters (radiators, baseboard, etc.) for 120°F or lower water delivery, and
- Mix down to deliver 120°F to the distribution (not strictly necessary, but a John Siegenthaler recommendation).
If you do nothing else, just do 1. Numbers 2 and 3 aren’t difficult with a bit of guidance.
Closing thoughts
Beyond dollars, cents, and COP, I’ll close with some thoughts on how this project has impacted our home. Simple payback be damned, this was a huge win for us. Why?
- We no longer have comfort issues in our home; in fact, we have highly tunable individual room control that simply works. It’s an amazing upgrade. People are surprised that we can get high quality heat with nothing more than 105°F water from the heat pump.
- We have no fossil fuels in our home—and thus a significant health and safety/risk improvement, which was very important to our family.
- We paired this installation with a high-efficiency (balanced) fresh air ventilation system—measurements of indoor air quality before and after is a whole different topic (but has had a big impact that I’ve quantified with data).
- Our first-floor slab heat is now balanced.
- Removing the boiler, removing the exhaust-only ventilation system, opening up and tightening the mechanical room, and filling all the passive air inlets in the home reduced our ACH50 to 2.1 (a 10-15% improvement).
- The heat pump water heater works symbiotically with the heating system, scavenging waste heat from the mechanical room slab, heat pump indoor unit, pumps, and buffer tank.
- Our mechanical room has become a nice cool/dry zone where we now hang laundry (which we have a lot of, in cloth diapering our twins).
How do you put a price on all of that? Simple payback is the wrong measure.
I was fortunate to be supported by great professionals, including an HVAC contractor who did a lot of learning on his own time rather than charging the customer for it. There are things I’d do slightly differently knowing what I do now (system and buffer tank size), and I’m also excited for 3-4 new air-to-water heat pumps scheduled to hit the market later in 2020 that have some intriguing features.
In the meantime, I’ll sign off with my favorite photos from the project.
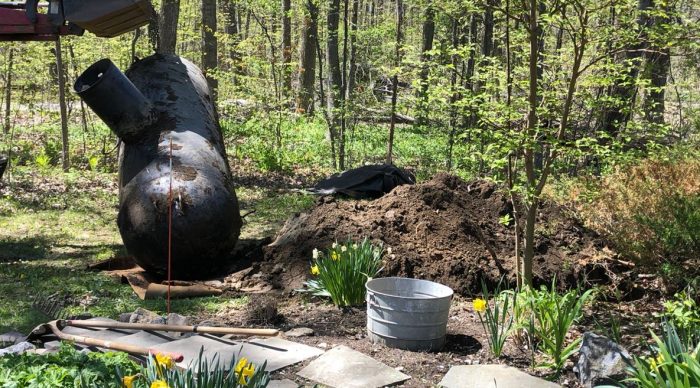
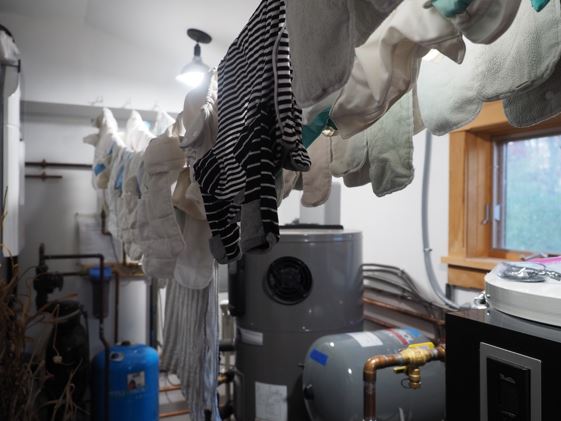
Originally published on GreenBuildingAdvisor.com. Brian Just is a mechanical engineer who manages a team of energy consultants at Efficiency Vermont. Photos and illustrations courtesy of the author.